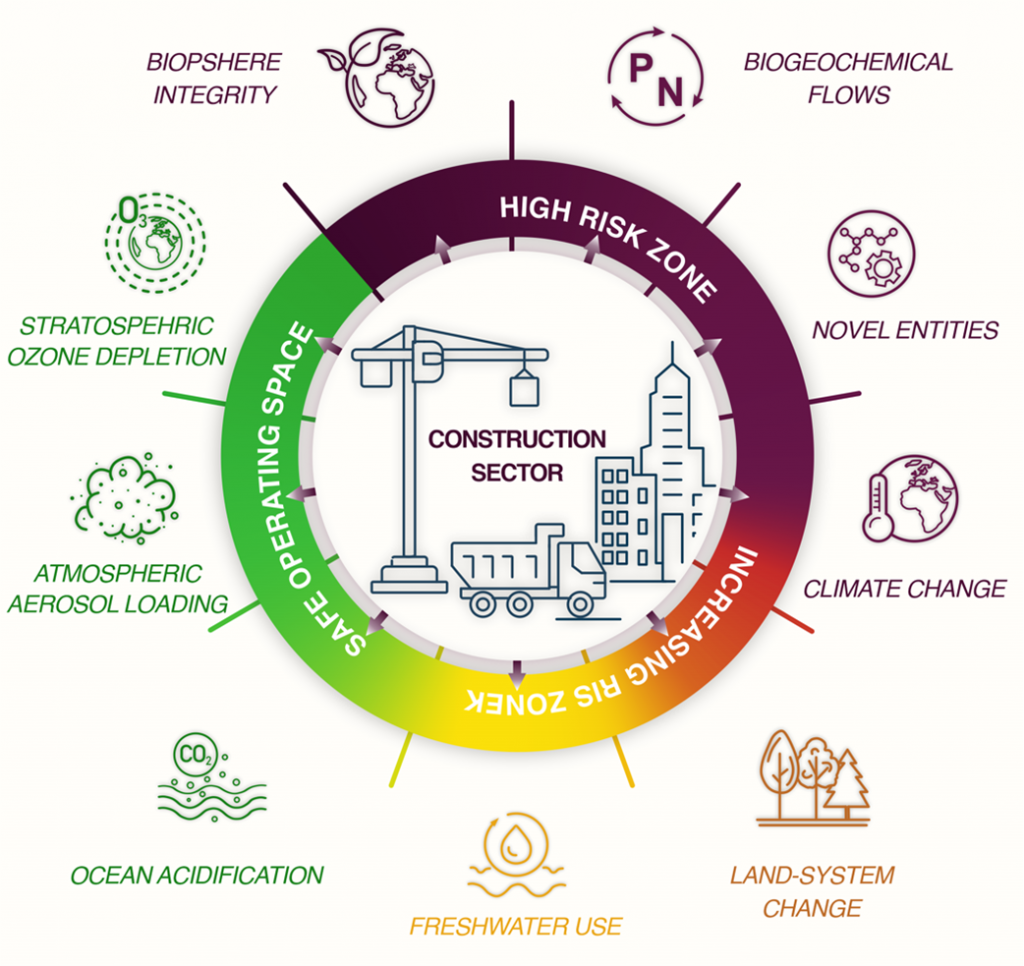
December 4, 2024
The European Union has implemented a range of policies across sectors, such as climate, energy transport, and taxation, to achieve a reduction in net greenhouse gas (GHG) emissions of at least 55% by 2030, compared to 1990 levels [1]. Programs like Horizon Europe, which support research and innovation aligned with the European Green Deal’s objectives, promote collaboration to address key environmental, economic, and societal challenges. These contribute to green building policy changes at the local, regional, or national level. Such actions aim to decarbonise and promote circularity in the built environment by advancing sustainable materials, thereby supporting global efforts to achieve climate neutrality.
Impact of the construction sector on the planetary boundaries framework
The planetary boundaries framework (PBF) was developed to define and characterise the thresholds of acceptable alteration across nine key Earth systems: biosphere integrity, climate change, ocean acidification, freshwater use, atmospheric aerosol loading, novel entities, biogeochemical flows, land-system change, and stratospheric ozone depletion [2]. These boundaries are not equally influential in sustaining or interacting with each other. Specific areas serve as leverage points where a single intervention could positively impact multiple Earth system components (Fig 1). These interactions fall into three categories; biophysically mediated and human-mediated interaction—further divided into parallel human drivers of change and reactive human-mediated mechanisms—offering substantial opportunities for synergies [3]. Enhancing air quality, improving soil health, creating carbon sinks, promoting circular resource flows, fostering biodiversity, and conserving water are key actions to maintain the PBF within acceptable limits [4].
Climate change, driven by elevated levels of CO2 and other GHGs, traps solar energy in the atmosphere, triggering cascading effects on all other planetary boundaries. One-third of global CO2 emissions stem from the manufacturing of building materials and building operations [5]. Developing sustainable construction solutions for urban areas is a key element in providing comfortable housing conditions for a growing population while adapting to climate change. In the 20th century materials like concrete, steel, and bricks dominated multistory residential construction. However, transitioning toward regenerative practices in the built environment can provide cascading advantages. Sustainable transformation in the residential construction sector closely aligns to the UN Sustainable Development Goals, particularly goal 9 (Build resilient infrastructure, promote inclusive and sustainable industrialization and foster innovation), goal 11 (Make cities and human settlements inclusive, safe, resilient, and sustainable), and goal 12 (Ensure sustainable consumption and production patterns). Within this context, biobased materials are evolving from an innovative alternative to becoming the “new normal.”
Fig. 1 Impact of the construction sector on planetary boundaries, categorised into high-risk, increasing risk, and safe operating space [6]
Materials have been fundamental to the progress of civilizations. Today, a material’s value depends on its purpose, properties, availability, cost, manufacturing process, safety, and environmental impact. Reducing embodied carbon involves minimising the use of virgin materials while considering their sourcing, extraction, processing, and end-of-life management in both new construction and retrofitting. Fast-growing species like bamboo and hemp present sustainable alternatives to timber, offering lower ecological footprints and greater carbon sequestration potential. Advances in engineered wood products, the use of underutilised wood species, and the incorporation of reclaimed wood further expand sustainable material options, reducing waste and improving resource efficiency.
To minimise water consumption in construction, materials should be selected based on low embodied water use. Similarly, aerosol pollution can also be mitigated by controlling particulate emissions during construction and demolition through strategies like deconstruction and component reuse. However, addressing “novel entities”, new substances, modified forms, and pollutants with potentially harmful effects remains complex due to a wide range of pollutants and their chemical interactions. Man-made building materials often generate pollution throughout their lifecycle. For instance, steel production emits significant pollutants, while materials like lead paint, asbestos, vinyl flooring, and flame retardants pose inherent toxicity risks. Humanity has already exceeded the planetary boundary for novel entities, necessitating a shift to non-toxic materials that safeguard both human health and ecosystems. Sustainable material choices in the built environment can play a pivotal role in reducing emissions and advancing ecological resilience.
Engineered living materials as new, resilient building components
Engineered living materials (ELMs) are emerging as transformative components in sustainable construction. By integrating biological elements, ELMs offer unique functionalities, such as self-repair and pollutant monitoring, surpassing conventional smart materials. ELM’s possess enormous potential as a new generation of active materials in sustainable built environments or as an integrated network of living sensors to monitor the presence and movement of pollutants and pathogens [7].
ELMs have the capacity to stimulate demand and create new market opportunities, allowing countless industrial and societal applications. However, their successful application requires addressing the ethical, legal, and social aspects, in addition to overcoming technical challenges related to design and upscaling of manufacturing processes. Social acceptance and trust are strongly tied to the approval, consent, and expectations from local communities and other stakeholders regarding specific materials or manufacturing processes. This includes demands for transparency, ethical considerations, and potential impact on health, the environment, or society. ELMs, which incorporate living cells of bacterial, fungal, plant, or mammalian origin, may be perceived negatively by the public. The presence of living microorganisms embedded in non-living scaffolds could discourage investor buy-in and customer adoption. Hence, targeted communication strategies about innovation, green technology, and novel properties are essential to foster understanding and acceptance.
Fostering Responsible Innovation and Adoption of Engineered Living Materials (ELMs)
While recent R&D efforts focus on designing, modelling, upscaling, and fabricating ELMs, it is equally important to address the ethical, legal, and social implications to create community-responsible innovations. These innovations aim to solve complex problems with the intent to deliver positive societal and environmental impact. As such, researchers should engage proactively and regularly with policymakers and the public. Identifying and communicating about the common barriers specific to the adoption of ELMs and future commercialisation with relevant stakeholders is crucial for achieving broad social acceptance. Since emerging technologies are usually more expensive, new environmental regulations (e.g. carbon tax) might enable ELMs to be more market-competitive [8]. Additionally, standardisation and the necessary legislation should be pursued by establishing strong partnerships with relevant partners.
A unique aspect of ELMs is their approach to material maintenance, which is in the form of nurturing and nursing living cells. This shifts the focus from conventional material preservation and repair to practices resembling farming, emphasising a shift from human-material interactions to human-material relationships.
Future perspective
The future of material science lies in creative and resilient designs that align with the PBF. As urban populations expand, cities must adopt low-carbon, sustainable materials to drive climate action and foster innovation, creating built environments that operate within the planetary boundaries. Materials scientists, designers, architects, and engineers are uniquely positioned to address the challenges of exceeding planetary boundaries. Cities as major resource sinks and sources of emissions, significantly influence global environmental outcomes. Consequently, the design, construction, and management of buildings play a critical role in shaping the future of both humanity and the planet.
References
Acknowledgments
This work was co-funded by the European Union (ERC, ARCHI-SKIN, #101044468). Views and opinions expressed are however those of the author only and do not necessarily reflect those of the European Union or the European Research Council. Neither the European Union nor the granting authority can be held responsible for them.
Authors: dr. Anna Sandak, dr. Karen Butina Ogorelec, dr. Anja Černoša, Ana Gubenšek, dr. Wojciech Pajerski, InnoRenew CoE